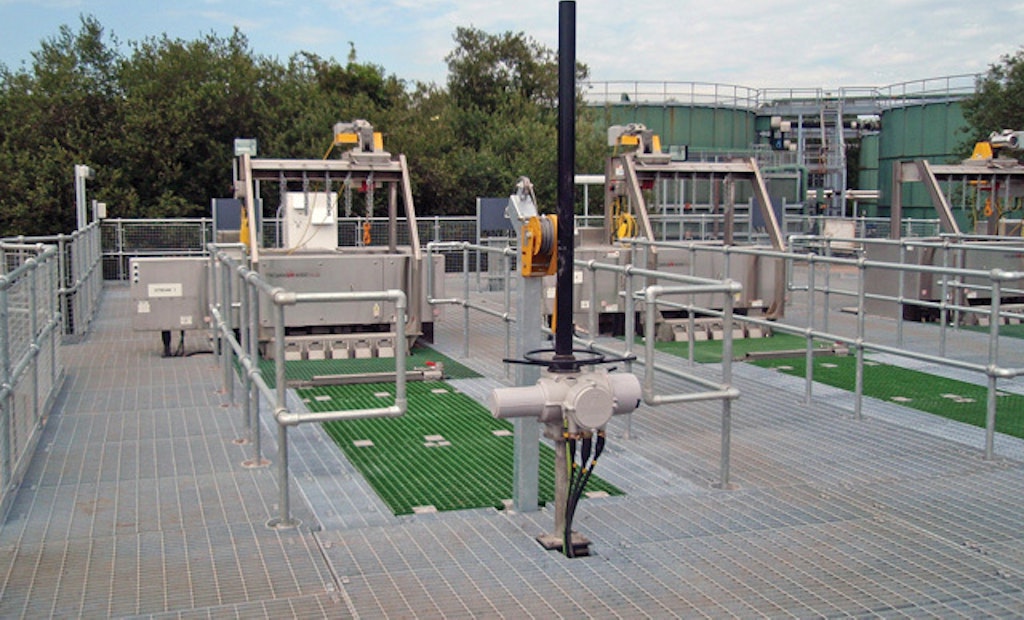
Ultraviolet (UV) light is used to disinfect many types of water, including low-quality water like primary treated wastewater and combined sewer overflows (CSOs). CSO treatment is becoming a regulatory requirement in many regions.
Chlorine is traditionally used to disinfect CSOs...